An international team led by chemist Tom Nilges from the Technical University of Munich and engineer Karthik Shankar from the University of Alberta have now found a stable yet flexible semiconductor structure that splits water much more efficiently than was previously possible.
An inorganic double-helix comprised of tin, iodine, and phosphorus (SnIP) forms the core of the structure, which is synthesized in a relatively simple process. The team prepared nanoparticles from each of the aforementioned starting materials and mixed suspensions of the nanoparticles with each other. The result was a structure with a hard but flexible SnIP-core and a soft carbon nitride shell.
The resulting SnIP fibers are flexible yet robust, with a Young’s modulus (relationship between stress and strain) along the double helix axis, which is comparable to steel. “The material combines the mechanical properties of a polymer with the potential of a semiconductor,” says Tom Nilges. “From this, we can manufacture flexible semiconductor components in a further technical step.”
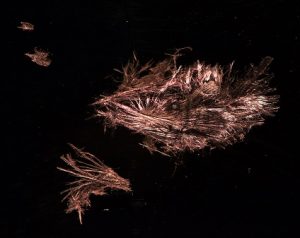
Needles of the flexible semiconducting SnIP. Image Source: A. Battenberg / TUM
Measurements show that the resulting heterogeneous structure is not only significantly more stable than the initial materials, but it was also shown to split water four times more efficiently than was previously possible, making it an interesting candidate for producing cheap hydrogen or to chemically store surplus electricity from wind farms.
To enhance catalytic efficiency, the researchers increased surface area by splitting the SnIP fibers into thinner strands. The material is in principle one-dimensional, with the thinnest fibers measuring a few nanometers thick and are comprised of several double helix strands. Wrapping it in carbon nitride allows the material to retain its high reactivity while becoming more durable – thereby making it more suitable as a catalyst. A mixture of 30% SnIP and 70% carbon nitride turned out to be the most effective combination.
These novel, one-dimensional SnIP double-helices open the door to different applications. The researchers indicate that future directions involve obtaining single strands of SnIP, which would be right or left handed and their own respective special optical properties. This makes SnIP a highly attractive material for optoelectronics.
“We were able to show theoretically that many other compounds of this kind are possible. Currently we are working on the synthesis of these materials,” says Nilges. “Flexible, inorganic, nanometer-sized, 1D semiconductors might create as much hype as 2D layered materials like graphene, phosphors, or molybdenum disulfide do today.”