The human body has the capability to regenerate, yet sometimes the site of damage does not have the right conditions for the body to begin reconstruction. Cell transplantation is a treatment that has the potential to offer permanent symptomatic relief for patients with a variety of diseases and injuries. Once injected into the site that needs repair, healthy cells with the ability to build new tissue can boost the natural regenerative process.
However, to date, inconsistent outcomes in preclinical and clinical trials have highlighted the need to advance cell administration strategies to improve and standardize the administration of cells. Moreover, researchers have observed major variations in cell survival and integration within the host tissue, which is attributable to a lack of a supportive regenerative environment and damaging conditions during implantation, but which also does hinder the translation of potential therapy into clinical practice. Most cells do not exist in isolation; instead, they are surrounded by a structure that supports, protects, and instructs them.
A group of scientists from the Australian National University and Deakin University is interested in developing materials to improve the efficacy of cell transplantation, help the cells integrate within the tissue, and enable the functions that will allow the technology to meet its full potential.
“To date, much improvement has been made to improve the differentiation protocols of transplanted cells to define their fate, with a combination of proteins and cells being implanted to attempt to improve their immediate survival within the tissue,” explains Professor David Nisbet, one of the study’s authors. “However, the efficacy of the technology remains affected by a critical need to improve the long-term survival and integration of transplanted cells. We, and others, believe that this is due to the lack of a carrier material to take on the protective role of the extracellular matrix.”
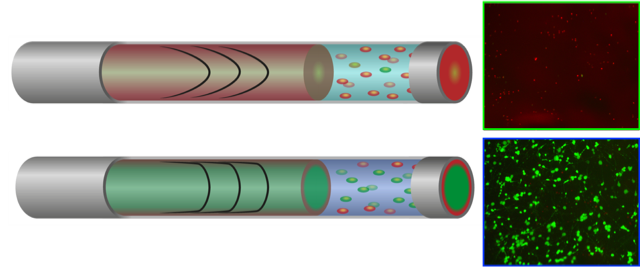
The authors believe that increasing the long-term survival of transplanted cells for repair after injury to the brain (and other tissue) will depend on an ability to provide a suitable milieu for the deployed cells to differentiate into new functional cells that can integrate within the host circuitry. This must involve the fabrication of synthetic, yet biologically relevant, cellular microenvironments that are capable of chemically and physically supporting the survival, differentiation, and integration of transplanted cells within the target tissue.
To this end, the researchers have engineered a delivery hydrogel that can be programmed with features that protects the fragile regenerative cells as it mimics the essential aspects of the native extracellular matrix. They use designed peptides to self assemble into a functionally rich, yet soft “safe sanctuary” microenvironment. Importantly, they present a mechanistic understanding of the multifactorial role of these nanoscaffolds as vectors in promoting cell survival and function, which could allow researchers to rationally design cell delivery vectors for their intended target tissue in the future.
Dr. Richard Williams summarizes: “We have isolated the key parameters of an ‘ideal’ cell transplantation material: i) physical support, ii) biologically active epitopes iii), ability to flow through a needle, and iv) active re-distribution of the shear stress generated during administration. Ultimately, we find that addressing each of these criteria in a single material significantly improved the survival of transplanted cells imminently following administration and in long-term culture.”
These peptide programmed hydrogels allow for the establishment of fundamental rules for the engineering of advanced treatment strategies with wide reaching implications for tissue repair, biofabrication, drug, and gene delivery and cell-instructive 3D culture.
“The study provides clinically relevant guidelines for the rational design of multifunctional hydrogels to improve cell transplantation, in this case the direct injection of neural cells, to promote their integration and long-term survival. As a step towards translation, we have commenced studies using our new delivery vector, transplanted neural progenitor cells within the brain (as proof of principle) to determine how improved cell survival and integration relates to functional recovery in animals….”, concludes Professor Nisbet.