The ancient Greek philosopher Aristotle, more than two millennia ago, recognized and attempted an early explanation of the unique feature of chameleons to switch their color from one hue to another [1]. Like many vertebrae able to make these impressive color changes to their skin for the purposes of communication, camouflage and thermoregulation, the origin of the color shift effect in the chameleon was generally thought to be through assembly-disassembly of organelle-containing pigments induced by neural or hormonal changes [2].

Photonic crystal chameleon – active photonic color from a tunable light-scale periodic structure – nature to laboratory to market
In a recent study [3] this picture of the variation of chameleon color has changed dramatically and has been traced as photonic rather than pigment color in origin, whereby the dimension of a photonic crystal comprised of a light-scale lattice of guanine nanocrystals is actively tuned to produce structural color variations. This bio-photonic color system is founded upon an upper dermal photonic crystal layer structured to reflect visible wavelengths of light, while a lower dermal layer is organized to reflect near infrared wavelengths, endowing the chameleon with predator protection, communication skills and body temperature control [3].
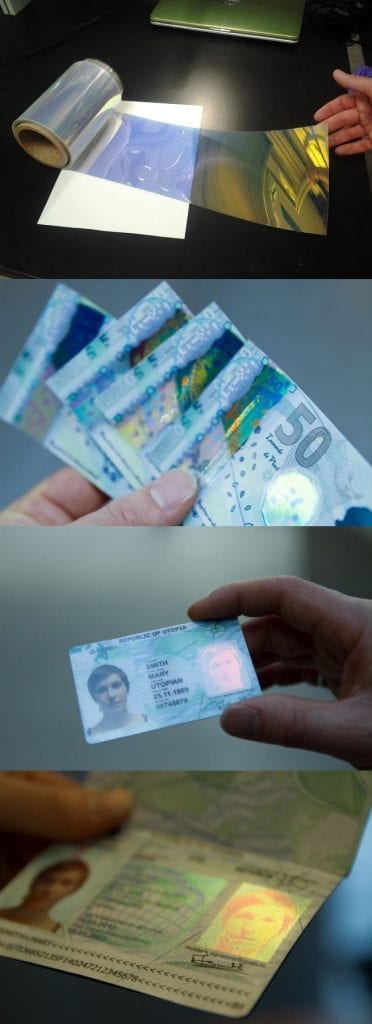
OpalPrint roll-to-roll manufactured photonic color base material fabricated into product specific security features on banknotes, identification cards and passports – courtesy of Opalux Inc.
Roughly two decades before the phenomenon of color shifting in the chameleon was recognized as originating from photonic structure changes rather than pigment effects, color from structure was being developed in the materials chemistry laboratory in both passive and active synthetic forms [4]. Two classes of photonic color emerged in this early research. One involved “passive” structural color, derived from the reflection of light from a photonic crystal with a static lattice, which offered a single reflected color, by Bragg diffraction, the wavelength of which depended upon the dimension and refractive index of the lattice. The other, photonic crystals displaying “active” structural color, by contrast uniquely provided any reflected color from the near infrared to the visible to ultraviolet wavelengths, by Bragg diffraction from a dynamic lattice whose dimensions and refractive index could be continuously tuned for example by electrical, mechanical, thermal and chemical stimuli [4].
This was the first time that a single material could present an infinite number of colors in a continuous color-shift mode, a feat previously only achievable by mixing the complementary colors, red, green and blue. It represented a laboratory demonstration of color changes in the chameleon before it was known that the color shifting in the chameleon was photonic!
Since that time photonic crystals have moved into the realm of 1D, 2D and 3D light-scale photonic lattices and their practice, often stimulated by theory, has transitioned rapidly from mainstream photonic science to advanced photonic technology [4]. There is ample evidence from published research that the ability of photonic crystals to control photons can rival that of electronic crystals to manage electrons. This has proven to be especially true for high refractive index contrast 3D photonic crystals made of silicon whereby an omni-directional photonic band gap offers complete control over the movement of light in all three spatial dimensions.
Today it has been said that ‘photonic crystals have been the classic underachievers: full of promise, sound in theory but poor on implementation’. This criticism has mainly come from the optical physics community because of the difficulties experienced of adapting top-down semiconductor engineering techniques to fabricate photonic lattices with high enough structural and optical quality to enable the scale-up, manufacture, economics and delivery of photonic crystal components and modules into markets that include solar cells, light emitting diodes, lasers, displays, sensors and optical fibres.
Observing that top-down approaches to the fabrication of photonic crystals often require complex and expensive lithographic instrumentation, deposition equipment and clean-room facilities, materials researchers realized there could be an easier way to make photonic crystals using bottom-up building-block assembly methods. Using this approach, the choice of polymers and materials that could be employed for the building blocks was vast. The variety of compositions and structures available for the building blocks enabled a cornucopia of photonic crystal classes to be synthesized offering a range of electrical, optical, magnetic, thermal, chemical, electrochemical, photochemical and catalytic properties that could be tailored to a desired function and perceived utility [4].
This is more-or-less how the self-assembly approach to making tuneable photonic crystals began and has blossomed forth today into one of the most scientifically vibrant areas of research with a multidisciplinary footprint that is envisioned to deliver manufactured photonic crystal products to diverse markets. These include sensors for food and water quality control to anti-counterfeiting devices on banknotes and passports, security features on consumer goods to displays.
About a decade ago, active photonic color research was transferred from the laboratory to the marketplace to create a new dimension in authentication technology [5]. Keeping good company with the chameleon, a wide range of attractive, customizable, counterfeit-resistant security features have been developed by Opalux Inc., which command attention and provide straightforward verification. These features include interactive security features, individualized optically variable portraits, RFID-activated tags and chemical sensors. These security features, being photonic crystal in nature, are very hard to duplicate and are easy to integrate with a variety of standard security printing production methods and materials. These chameleon-like security features are bright, stable, durable, customizable and available in a wide variety of appearances and performance characteristics.
This class of tunable photonic crystal security features with bright unique chromatic appearances, like the chameleon have a precise nanostructure which makes them optically variable. Their appearance changes when viewed at different angles and under different lighting conditions. For example, transitions between bright reds, yellows, greens and blues, or even ultraviolent and infrared, are possible as lighting and viewing angles change. These colors are tunable, meaning that their appearance changes in response to a range of stimuli. Four photonic color technology platforms that provide powerful security features based on thermal, mechanical, electrical and chemical activation include OpalPrint, ElastInk, Photonic Ink and Photonic Nose [5].
OpalPrint is permanently patterned by the application of laser or heat. It can be used to provide fully customized and personalized optically variable devices, like optically variable passport holder portraits. OpalPrint technology combines personalization with optical variability in a single security feature to create personalized security. It provides attractive, optically variable document holder portraits for passports and identification cards. It can also provide other helpful authentication information, like signatures, control numbers and biometric information in a secure, easily read, optically variable feature. Personalization of OpalPrint film is accomplished by laser tuning. Because OpalPrint is compatible with a range of security industry-standard laser personalization techniques it can be personalized both in centralized and decentralized production environments. OpalPrint can be used with a variety of security substrates, including paper, polymer and polycarbonate.
ElastInk is Opalux’s interactive security feature. In addition to its inherent optical variability, ElastInk changes the information content in response to the application of finger pressure [6]. The pressure threshold, duration of activation and other aspects can all be customized. Elastink can be produced in a wide variety of form factors, including as a security thread for paper banknotes. This latter variant meets circulation hazard requirements for paper banknotes. Depending on security requirements, Elastink’s appearance change can be either reversible or irreversible. For example, when used as a security thread in a banknote, a reversible variant is employed. However, for other applications such as tamper evidence, irreversible variants and variants that reverse in response to specific stimuli like heat may be employed. ElastInk is available in a range of initial colors, including red, yellow, green and blue, and a variety of pressure thresholds. Several variants of ElastInk are reserved for high security documents.
Photonic Ink is an RFID-activated security feature. Photonic Ink dramatically changes its appearance in response to the presence of an RFID field or other sources of electrical charge [7] and is optically variable. Photonic Ink operates at low voltages and current and can maintain static images with negligible energy. Photonic Ink can be deployed on rigid and flexible surfaces, is stable and not affected by harsh environments.
Photonic Nose authenticates products quickly, reliably and inexpensively by changing appearance in response to a variety of chemicals [8]. The human nose works by using a large array of olfactory neurons, each responsive to a different stimulus. By working in combination with each other, this array of olfactory neurons detects a wide range of substances, quickly, reliably and inexpensively. Inspired by the human nose, Photonic Nose uses an array of photonic crystal sensing units. These sensing units, in conjunction with a digital camera and image analysis software, permit the detection of a wide range of substances. Simpler versions, which change appearance in response to simple stimuli like water vapor, are also possible.
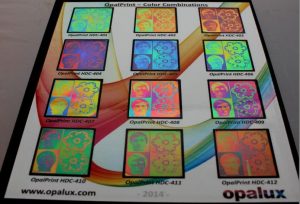
OpalPrint color combinations – courtesy of Opalux Inc.
It is interesting how about two decades before it was known that the color shifting in the chameleon was photonic, the physical, chemical and biological principles that underpin the chameleon’s skin color altering ability were being used in the materials chemistry laboratory to develop color from structure and have now been adapted and scaled in the advanced materials science and technology world in order to manufacture multi-color security devices that include banknotes, credit cards, passports, driving licenses, identification cards, tax stamps, color changing surfaces and graphics, automotive applications and smart windows [5].
[1] Anderson, C.V., Historic and contemporary theories on chameleon color change. http://www.chameleonnews.com/04NovAndersonColor.html.
[2] Nilsson Skold, H., Aspengren, S., Wallin, M., Rapid color change in fish and amphibians – function, regulation, and emerging applications, Pigment Cell & Melanoma Research, 2013, 26, 29-38; Taylor, J. D., Hadley, M. E., Chromatophores and Color Change in the Lzard, Anolis Carolinensis, Zeitschrift fur Zellforschung und Mikroskopische Anatomie, 1970, 104, 282-294.
[3] Teyssier, J., Saenko, S.V., van der Marel, D., Milinkovitch, M.C., Photonic crystals cause active color change in chameleons, Nature Communications, 2015, DOI: 10.1038/ncomms7368.
[4] von Freymann, G., Kitaev, V., Lotsch, B., Ozin, G.A., Bottom-Up assembly of photonic crystals, Chem. Soc. Rev., 2013,42, 2528-2554; DOI: 10.1039/C2CS35309A.
[5] www.opalux.com.
[6] Arsenault, A.C., Clark, T.J, von Freyman, G., Cademartiri, L., Sapienza, R., Bertolotti, J., Vekris, E., Wong, S., Kitaev, V., Manners, I., Wang, R.Z., John, S., Wiersma, D., Ozin, G.A., Elastic Photonic Crystals: From Color Fingerprinting to Control of Photoluminescence, Nature Materials 2006, 5, 179-184.
[7] Puzzo, D., Arsenault, A.C., Manners, I., Ozin, G.A., Full Color Photonic Crystal Display, Nature Photonics 2007, 1, 468-472.
[8] Bonifacio, L.D., Puzzo, D.P., Willey, B.M., Kamp, U., McGeer, A., Ozin, G.A., Towards the Photonic Nose: A Novel Platform for Molecule to Bacteria Identification, Adv. Mater. 2010, 22, 1351-1354.