The authors R. Xue, M.A.B. Baker and F. Bai summarize in their progress report paper all known information regarding structure, assembly, torque-generation, switching, and adaptation properties of bacterial flagellar motor.
The team used Escherichia coli as model organism to explain the highly ordered structure and the organization of the bacterial flagellar motor. E. coli has about 4-5 flagella randomly distributed on the cell surface. Each flagellum consists out of three clearly distinct compartments, namely flagellar filament, hook and flagellar motor.
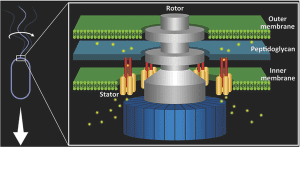
Rapid rotation of the flagellum is driven by the bacterial flagellar motor embedded in the cell envelope, harvesting the free energy of ion flux across the cytoplasmic membrane.
The flagellar filament is a long helical hollow tube. The conformational change of the filaments results in a change of the rotation direction which leads to a run or tumble movement of the bacteria. The filament is connected to the motor via a flexible hook. The hook is a short curved tube, where the curvature stays unchanged during flagellum rotation. This allows a highly efficient torque transfer from the motor to the filament. The flagellar motor spans across the outer membrane and is the central part of the flagellar. It consists of 20 proteins and has a molecular mass of about 11 MDa. The bacterial flagellar motor is a stepping motor and can rotate in two directions, counterclockwise (CCW, run movement) and clockwise (CW, tumble movement). With these two operational modes, the bacteria are able of chemotactic navigation. The bacterial flagellar motor has to deal with thermal fluctuation while still precisely coordinates the motion of multiple proteins. All the required energy is released by the release an ion flow down an electrochemical gradient across the cytoplasmic membrane into the cell (protonmotive force). The authors highlighted that there is a huge effort to determine the torque-speed relationship, a measure of the performance of any motor. Experimental studies indicate that the energy conversion efficiency of the bacterial flagellar motor is very high. Nearly the complete energy released by the protonmotive force is converted into mechanical rotation.
The bacterial chemotaxis means that the cell responds to temporal changes of attractant concentrations in its environment. This is achieved by the bacterial flagellar motor quickly switching between the CCW and CW stage. The bacterial surface contains chemoreceptors to detect the attractants. A signaling molecule becomes phosphorylated and the change of the phosphorylated cytoplasmic concentration then causes the rotation to switch between CCW to CW. The bacterial flagellar motor is remarkably sensitive and already small changes of the phosphorylated protein concentration result in conformational changes and so in a change of the direction of the rotation within milliseconds. The sensitivity can also be adapted due to structural adaption of the bacterial flagellar motor by e.g. changing the number of a bacterial flagellar motor protein.
The authors show clearly that the bacterial flagellar motor is the pinnacle of evolutionary bionanotechnology. By now no known artificial machine can reach the energy conversion efficiency and sensitivity of this bio system. However each further progress in analyzing and understanding this highly complex system will help to develop better nanotechnology in the future.