Image credit: Markus Spiske on Unsplash
Regulation of body temperature or thermoregulation is an important function and is vital for maintaining health. In mammals, various temperature-controlling biological mechanisms are crucial for sustaining thermal equilibrium, i.e., the balance between the rate of heat production and the rate of heat loss, for which countless organisms have evolved characteristically flexible mechanisms and behavioural adaptations.
Deviations from normal core body temperatures are in general harmful, but there are also circumstances in which they can be beneficial. For instance, an elevated body temperature during fever can help fight off pathogens. In a clinical setting, the precise regulation of body temperature in the form of targeted temperature management is an instrumental part of hospital intensive care. Lowering the body’s core temperature to 32-34 °C (mild hypothermia) to counteract severe hyperthermia that develops after successful resuscitation from cardiac arrest, for example, has been a part of therapeutic guidelines for almost two decades and has helped save many lives. Mortality rates improve with thermoregulation in these patients. The therapies also provide better neurological outcomes by protecting the brain against lack of oxygen and reduced perfusion.
While potentially lifesaving, inducing changes in body temperature in a clinical environment is difficult and associated with many secondary changes in physiology that can be detrimental, such as a profound lowering of the heart rate, increased urine output, and changes in electrolyte concentrations. With temperature management also come numerous additional therapeutic and diagnostic procedures (e.g., emergency coronary catheterization, CT scans, insertion of vascular catheters), all of which are time sensitive.
Additional challenges arise from variability in patient response as well as the fact that a variety of different methods to achieve temperature reduction exist, such as intravenous infusion of cold fluids, cooling blankets, endovascular cooling catheters, among others. Each of these has its benefits as well as drawbacks but predicting how a patient will respond and what the best course of action is can be difficult to predict. Therefore, it is of utmost importance to find new and even better temperature management techniques.
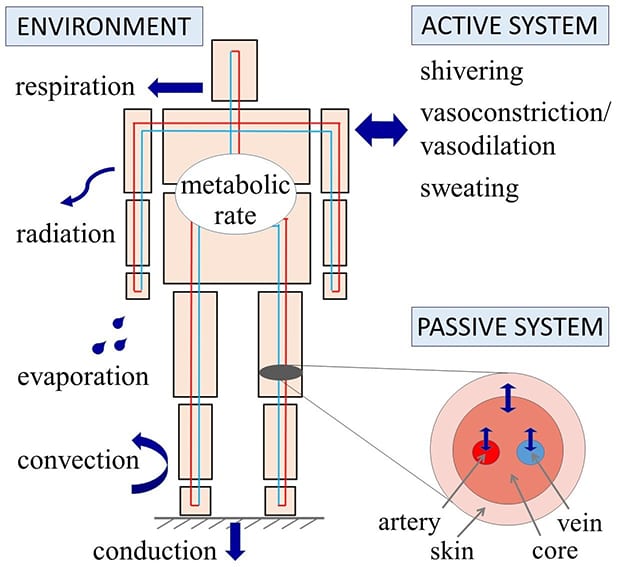
In recent years, computational bioheat models have been proposed to better understand the underlying bio-thermal processes and to predict changes in a patient’s thermal state. In these models, the human body is typically represented by two interacting systems of thermoregulation: the controlling active system, which represents the human body’s regulatory responses (e.g., vasoconstriction, vasodilation, shivering, sweating, and metabolic heat production) and the controlled passive system (e.g., thermal interactions between the body and the environment).
Many models available today are based on a composite model of the human body that consists of several cylinders representing the head, the corpus, and the upper and lower extremities. Heat exchange occurs between different body segments via blood flow and also within the segments by means of different heat transfer processes between the core, skin and blood.
Biothermal models of the human body are becoming increasingly comprehensive and an ambitious goal would be to combine a real-time and easy-to-use measuring device with a computational thermal model that is tailored to individual patients and can be used to predict and precisely regulate patient’s temperature changes during a hospital stay. The hope is that they will also aid in the design of special-purpose devices to control the delivery of thermal energy to targeted regions and to improve the treatment of diseases such as the delivery of therapeutics in cancer patients. This method is also known under the name temperature-controlled drug release.
One potential and promising example is the release of molecules from mesoporous silica nanoparticles that can be intravenously administered and react to an external heat stimulus (e.g., magnetic field). Especially in combination with advances in smart bio-measurement technologies, such interdisciplinary approaches have great potential for optimizing temperature management strategies in a variety of clinical settings. This is another example of how interdisciplinary endeavors at the interface of physiology, clinical research, biometrics, and biophysical modelling can lead to novel and innovative solutions.
Written by:
Kristijan Skok, General Hospital Graz II, Location West, Institute of Pathology, Göstinger Straße 22, 8020 Graz, Austria and University of Maribor, Faculty of Medicine, Taborska ulica 8, 2000 Maribor, Slovenia
Maja Duh, University of Maribor, Faculty of Natural Sciences and Mathematics, Koroška cesta 160, 2000 Maribor, Slovenia
Andraž Stožer, University of Maribor, Faculty of Medicine, Taborska ulica 8, 2000 Maribor, Slovenia
Andrej Markota, University of Maribor, Faculty of Medicine, Taborska ulica 8, 2000 Maribor, Slovenia and University Medical Centre Maribor, Medical Intensive Care Unit, Ljubljanska 5, 2000 Maribor, Slovenia
Marko Gosak, University of Maribor, Faculty of Natural Sciences and Mathematics, Koroška cesta 160, 2000, Maribor, Slovenia and University of Maribor, Faculty of Medicine, Taborska ulica 8, 2000 Maribor, Slovenia
Reference: Kristijan Skok et al., A Journey from Physiology to Computational Models and the Intensive Care Unit, WIREs Systems Biology and Medicine (2020). DOI: 10.1002/wsbm.1513