In some countries the most energy-efficient and cost-effective way of storing excess electricity, whether fossil or renewable on a large scale, greater than Megawatts, is in the form of potential energy by pumping water up to mountain lakes, as thermal and mechanical energy by adiabatically compressing air, and as rotational energy in spinning flywheels. In many countries, all of these electricity storage methods combined represent only a few percent of actual total electricity generation.
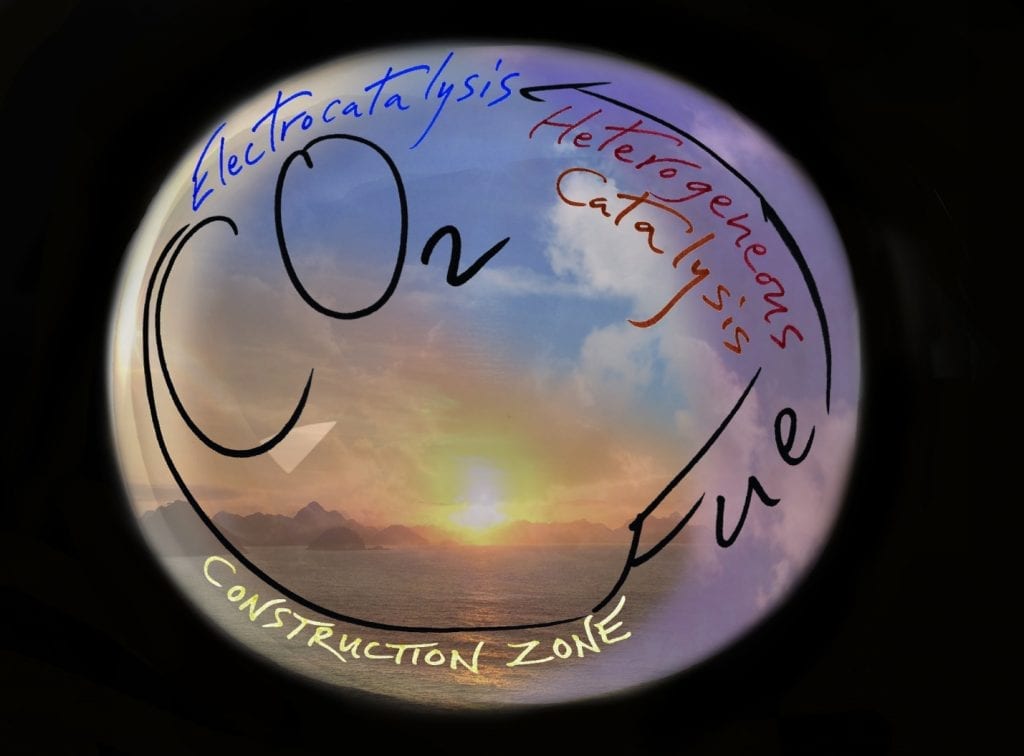
Artist Todd Siler’s metaphorm of “The Big Picture of World War III on Climate Change” being fought between two current competitive approaches to converting CO2 to Value-Added Chemicals and Fuels, in a carbon-neutral carbon-cycle. Intimated here is the possibility that the “Construction Zone” that enables sustainability is our oceans and seas that are central, or core, to the whole system of conversion. The “L” letter in the word “Fuel” is shaped so it reads like a vector, making one wonder if these two competing systems of CO2 utilization catalysis are more complementary than we presently understand them to be, www.artnanoinnovations.com.
There are many opinions on this subject and until grid-scale storage of electricity, in for example flow batteries, is part of the electricity infrastructure the jury is still out on what to do with excess electricity, especially as renewable electricity becomes abundant, the essence of our envisioned sustainable future. An important point here is that flow batteries are good for diurnal storage, or at best short term storage of electricity. This is due to limited capacity and temporal discharge. Chemical storage of electricity on the other hand using electro-catalysis or heterogeneous catalysis methods allows seasonal storage, which can offer advantages for example, in electricity rich provinces, making use of electricity for the production of chemicals and fuels. Depending on location, chemical storage may be best in the summer for use and consumption in the winter or vice versa.
A contemporary view is that electrochemical storage of excess electricity by converting an aqueous solution of CO2/H2O into chemicals and fuels by electro-catalytic methods is a good strategy for fighting climate change. A parallel opinion is that the excess electricity can be used to drive thermochemical transformations of CO2/H2 by heterogeneous catalytic approaches to chemicals and fuels. The idea in both cases is that grid-scale electrical-to-chemical energy storage can be employed to control load leveling of power and demand of the electrical grid as well as the production of value-added chemicals and fuels. A counterargument to this approach centers on the thesis that currently there is not enough excess electricity to have any significant impact on climate change and instead the surplus is more wisely used in charging stations to power up the rapidly growing number of electric vehicles. Some believe that charging stations directly powered by solar electricity may be the way of the future.
Others believe in a much grander plan – an international effort to provide renewable electricity to 139 nations around the globe by 2050 with about 37% wind, 58% solar, 4% hydropower, and 1% geothermal, wave, and tidal power. This would essentially eliminate the need for stationary storage batteries, biomass, nuclear power, carbon capture, or natural gas.
While all of this may be good for urban transportation, it is not feasible for long-haul road and aviation mobility, where high energy density fuels are needed. This may change with time, imagine short flight electric and long haul fuel cell planes, however there will be a pretty long dependence on hydrocarbon transport fuels. Electricity to chemicals and fuels technologies by electro-catalysis and heterogeneous catalysis offers a potential solution. While at first glance this appears to be contrary to mitigation strategies, as CO2 is released on combustion, an often neglected fact is that by using this approach it is possible to avoid more fossil oil-derived fuels entering the supply chain.
Another concern is the question of the cost of electricity, which is the most expensive ‘reagent’ for converting CO2/H2O and CO2/H2 into stored chemical energy using aqueous phase electro-catalysis or gas phase heterogeneous catalysis, respectively. If one could do this using sunlight directly, it becomes a non-issue, but neither of the solar powered CO2 to chemicals and fuels processes are efficient enough processes to be commercially viable.
So, the cost of electricity is a key parameter in any business model for making a profit from CO2 and is being investigated extensively. The challenge for electrochemical synthesis using CO2/H2O as the feedstock, at today’s industrial electricity rates, is to make the product at a competitive cost. Therefore, to achieve commercially acceptable energy and economic flows, together with an overall carbon neutral footprint, one needs to pick a product carefully with respect to the lowest possible electricity load and highest value-added product, as well as choosing ones energy source carefully in terms of cost. The jury is still out on the product of choice for a commercially successful business.
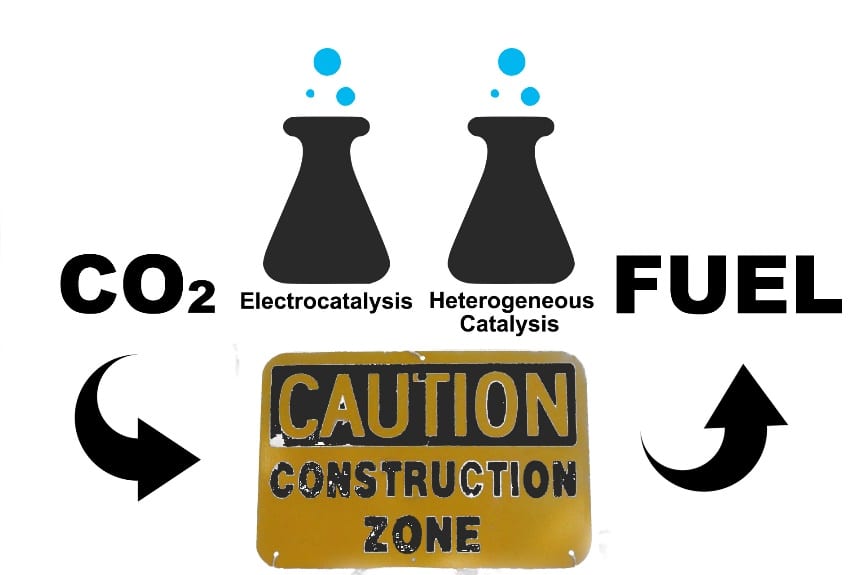
In the war against climate change two promising approaches are being developed for catalytically converting CO2 to value-added chemicals and fuels
It could be formic or oxalic acid as a safe hydrogen storage source for use in H2/O2 fuels cells, carbon monoxide as a feedstock for the synthesis of hydrocarbons and methanol, ethylene glycol for making polyester fibers and antifreeze, or ethylene as a basic building block for polymers and copolymers.
This is potentially good news for renewable electricity from wind and hydro during off-hour periods when the cost of the electricity is not too high. Solar electricity can work too if the chemical product is considered as a stored energy source for dark usage, but it is not commercially competitive with wind and hydro-electricity at this point for chemical synthesis using CO2 as the feedstock.
Here, one must question whether the development of new electrochemical technologies for the transformation of excess electrical energy to stored chemical energy could really have an impact on greenhouse gas climate change? This is an argument for integrating electricity powered, thermally driven heterogeneous catalytic reactor technology into the existing chemical and petrochemical industrial infrastructure. An alternative approach would be to integrate a small chemical plant next to a power plant. Capturing and transporting/storing CO2 can be expensive. A detailed techno-economic analysis is needed of course to decide which is better
In this way one can convert CO2/H2 to chemicals and fuels, at a sufficiently large scale, high efficiency and low cost that it could meaningfully impact climate change. Now the question of the source of H2 and its cost becomes a discussion point, just as one appraises the supply, demand and sources of electricity in different countries, the insolation that reaches the earth’s surface in different parts of the globe, and the availability of sufficient quantities of water of the right quality. Think about the surfeit of hydroelectricity in Quebec and Iceland and plethora of sunlight in the Middle East and North Africa.
In the context of countries rich in coal and natural gas that decide to keep on using them as an energy source, for example in electricity generating stations, an argument forwarded for their continued use, is that the heat of combustion can be usefully employed to drive gas-phase heterogeneous catalytic processes for converting the associated CO2 emissions to value-added chemicals and fuels. While this can be considered a short term solution, it wouldn’t work in the long term of achieving carbon-neutrality as the mined coal and natural gas will have to end up somewhere, which is likely the atmosphere in the form of CO2. The same specious argument can be voiced against the use of captured CO2 for enhanced oil recovery. On the other hand, renewable energy powered electro-catalytic and heterogeneous catalytic CO2 utilization processes can create a complete carbon-neutral cycle and hence a better long-term solution to climate change.
Perhaps a more important consideration would be the efficiency of multiple processes in the CO2 to chemicals and fuels supply chain. While storing grid electricity in batteries and charging units is probably more efficient than converting the electricity into chemicals and fuels using CO2/H2O and CO2/H2, and then using them later, there are all the other considerations that have to be taken into account. Both positive and negative, these considerations include the efficiency and scale of the conversion process, being able to store the chemicals and fuels for longer time frames and transport them across longer distances, and energy density in chemicals and fuels compared to batteries.
Since batteries are a well-developed and mature technology, while electro-catalysis and heterogeneous catalysis for CO2 conversion are still in their infancy, with none or very few commercial examples, one can understand the argument of storing excess electricity in electric cars. However, batteries cannot be used for large scale transportation such as tankers and cruise ships, planes and large trucks, which account for 40% of the transportation industry. So chemicals and fuels from CO2 may well play a part in this user sector.
In the case when CO2 derived chemicals and fuels are needed, whether electro-catalysis or heterogeneous catalysis is the better approach for their production is currently a very difficult question to answer, because there are so many factors that need consideration. For the case of electro-catalysis, one needs electricity to drive the kinetics and thermodynamics of the reaction. An economically viable aqueous phase electrochemical CO2 utilization technology will require a large current density to enable a high rate of conversion, a high Faradaic efficiency to favor a desired product, low over-potential to facilitate a high energy efficiency, and optimum electro-catalyst stability to permit a long electrode lifetime. Similarly, a practical gas phase heterogeneous catalytic CO2 conversion process driven by electrical energy should achieve high reaction rates, turnover frequencies and efficiencies under as mild conditions of temperature and pressure as possible. In both approaches, selective conversion of CO2, for example to CO, CO/H2, CH4, HCO2H, C2H4, C2H4(OH)2, CH3OH, and (CH3)2O is of particular interest. Providing there is sufficient electricity from the grid, whether derived from nuclear, wind, solar, tidal, geothermal or hydroelectric sources, and these chemicals are not more costly to produce by CO2 reduction than by current means, even with CO2 at $50/ton, this may be feasible.
Something to consider here is that industries requiring large amounts of energy tend not to be reliant on the grid. Instead they have their own cogeneration facilities, a combined heat and power system that provides both electric power and heat from a single fuel source. It may be that these industries will install wind turbines and solar arrays dedicated to the processes, offsetting the capital expenditure costs against buying in power. A problem here, is that these industries rely on a “steady” supply of energy because they tend to employ continuous “steady-state” units. So, they can use renewable technologies but they will still need to have “traditional” on-site production or be connected to the grid.
In the case of heterogeneous catalysis, at the moment there is no technologically significant, commercialized working version of CO2 + H2O conversion to chemicals or fuels. In most of this research, the reductant is H2, the majority of which comes from steam methane reformation and hydrocarbon sources. To make H2 renewably and reduce CO2 through heterogeneous catalysis in a carbon neutral carbon cycle, the best source would be through water electrolysis, which would need electricity anyway. So if both heterogeneous catalysis and electro-catalysis processes need electricity, is there really an advantage to one or the other? In this context, it is noteworthy that proton exchange membrane electrolysers for H2 generation have been commercialized where H2 is generated at the point of need.
The other considerations are infrastructural in nature. Where would electrochemical cells be located – in line with factories or refineries, downstream from various industrial processes, and how would CO2 be delivered to them? One advantage of electro-catalysis is being able to separate the electrodes and hence the products into different compartments. However, the aqueous-phase electrochemical systems are likely not as versatile and easy to implement as gas-phase heterogeneous catalytic systems, which are pervasive at the Megaton scale in the chemical and petrochemical industries. They can also be implemented as micro-structured heterogeneous catalytic reactors, modules of which can be assembled at different scales on-demand. Micro-heterogeneous catalytic reactors have the advantages of high energy efficiency, conversion rates and yields and provide scalable on-site demand and production with impressive process control. The analogue in electrochemistry would be microfluidic electrochemical cells, assembled to the scale required for a particular application and geographic location.
Electrochemical cells need a lot of infrastructure, including cells, electrodes, membranes and electrolytes. Problematical also is the low solubility of CO2 in water that can limit conversion rates to products and which have to be purified by separation from the aqueous phase. To overcome solubility challenges, specially designed porous electrodes are needed to enhance the interaction between the gaseous CO2, electro-catalyst and liquid electrolyte, thereby reducing mass transfer, charge transport, ohmic resistance and wettability restrictions in the electrochemical reduction of CO2. By comparison, gas-phase heterogeneous catalysis would need flow reactors, high pressures and high temperatures, and if solar powered, light harvesting.
So to really compare and decide which is preferred over the other, one needs a full energy, scalability and stability CO2 foot-print, and economic analysis, to meaningfully assess all of the aforementioned considerations. In this context, a number of life cycle and techno-economic analyses have been carried out in recent years. These show that the processes are currently not economically viable, however, this is mostly due to geographical location and economies of scale.
The reality today is that carbon capture and utilization technologies are small in comparison to global total carbon dioxide emissions and they are still two orders of magnitude greater than carbon capture and storage. Carbon avoidance is probably a better target at this time than focusing exclusively on mitigation.
My view is that collaborative research and development projects between universities and industry that focus attention on both approaches for converting CO2 to chemicals and fuels should be greatly intensified so that we have as many weapons of mass construction to support our war against climate change. The element of competition in research and development between the two approaches must be considered a good thing, assuming there are enough teams involved in each approach.
It is interesting to speculate what drives the research and development for each approach. Is it the commercial, technical and infrastructural considerations of the industrial companies which would adopt one of these approaches? There have been many inventions where there have been two competitive options but even though one was clearly technically superior to the other, the inferior one was adopted as the one throughout the industry.
At this stage, research and development for both approaches should be intensified if that is at all possible. Also it should be remembered that research and development does not move in a straight line and that if one of the processes is discounted at some point, there is a possibility that exciting developments for that approach could be lost.
In this context I am encouraged to see a number of heroic scientists, engineers and investors creating spin-off companies dedicated to the development of high efficiency and cost effective electro-catalytic and heterogeneous catalytic processes for converting CO2 into value-added chemicals and fuels. These include Liquid Light, Dioxide Solutions, Audi-Sunfire, Sandia National Laboratory, NewCO2Fuels, Solar-Jet-Fuels, Syntrolysis Fuels, Doty Energy-WindFuels, Air-Fuel Synthesis, Green-Feed, Opus 12, Enerkem, Quantiam, LanzaTech, Catalytic Innovations and E-Diesel.
Hopefully these CO2 utilization ventures will be successful in their goal of converting CO2 to profit and expanding their production facilities across the globe to a scale that can meaningfully impact greenhouse gas induced climate change.
On this Utopian vision, a knowledgeable colleague commented that to make a profit out of CO2, one has to find the right products. The end game most likely is not going to be the vector sum of many smaller commercialization vectors. Each one might point in slightly different directions, but together they will bring technologies that are massive and competitive enough to move the needle on CO2 emissions and climate change. Nobody currently knows what these paths will look like definitively, but I’m guessing the people who created the Apple App store and Google Play also did not predict Uber/Lyft and the many other billion dollar companies at the time.
In closing, maybe it is worth commenting that making a profit out of CO2 will always be difficult, as almost everything that can be made from CO2 can also be made from CO. Since syngas CO/H2 can be obtained from natural gas, it will be very difficult to compete. So maybe a better case can be made on societal need to address environmental challenges without too large a cost.