Reprinted/adapted with permission from Semenok, D., et al. J. Phys. Chem. Lett. 9, 1920–1926. Copyright (2018) American Chemical Society
The electrical resistance of a material stems from the scattering of current-carrying electrons by phonons. By coupling the electrons as pairs to the phonons the scattering process responsible for resistance can be eliminated. This is the essence of the 1957 Bardeen, Cooper, and Schrieffer (BSC) model of superconductivity, namely, resistance-free electrical conductivity enabled by renowned Cooper pairs.
The possibility of a room temperature superconductor was first injected into the psyche of science in 1964 by William A. Little, who imagined Coulomb-bound electron-hole pairs in conducting organic polymers, known as excitons, could also mediate electron pairing.
While superconductors are not considered an energy material, the energy savings arising from resistance-free transmission and distribution of electricity are potentially massive when considered on a global scale. In fact, the practical realization of room temperature superconductivity has been estimated to reduce energy losses in copper and aluminum cables and transformers by 7% in world-wide electric power transmission and distribution systems.
Energy could also be saved by incorporating room temperature superconductors into electricity generating power plants, storing electrical energy as persistent currents in superconducting magnetic loops, employing magnetically levitated railways as an alternative to air and road transportation, using superconductor propulsion motors for maritime transport, and improving the energy efficiency of quantum computers with superconducting Josephson junction digital logic circuitry. If room temperature superconductors would become a reality, the energy savings would be a significant enabler of the energy transition to a sustainable future.
The field of superconductivity has a long and fascinating history that emerged with the 1911 discovery by Heike Kamerlingh Onnes who observed that mercury loses its electrical resistance at liquid helium temperatures, known as the critical temperature Tc.
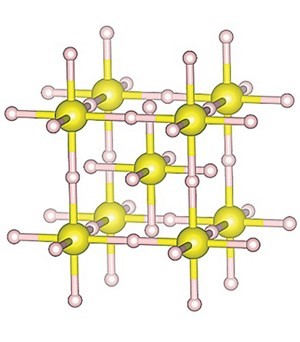
An explosion of activity in the search for superconducting materials bearing higher critical temperatures Tc ensued, and intense activity in the field today continues to seek out the holy-grail material that displays superconductivity at room temperature and ambient pressure.
With many different classes of inorganic and organic contenders competing in the race for the fabled room temperature superconductor, there exists a massive chemical space of combinations of the elements of the periodic table to work with. The discovery of the high Tc lanthanum copper oxide and yttrium barium copper oxide ceramic materials class of superconductors represented a milestone that greatly boosted optimism that a room temperature superconductor was a realistic goal, although with this class of materials, the quest seems to have stalled with the Tc = -140°C record ambient pressure superconductor HgBa2Ca2Cu3O8+x.
In the continuing search for higher Tc superconductor, materials enthusiasm was rekindled by the inspiring prediction of Neil Ashcroft in 1968 that hydrogen atoms under pressure would be metallic and exhibit superconductivity at room temperature or higher. While the high pressures to achieve this metallic hydrogen phase seemed unachievable, Ashcroft made another prediction in 2004 that metal hydrides could behave similarly, and the search accelerated again.
The rationale for Ashcroft targeting metal hydrides as high Tc superconductors is based on three key principles of BSC theory that pinpoint high Tc materials having high phonon frequencies, high electron density of states near the Fermi energy level, and strong electron-phonon-electron coupling to favor Cooper pair current carriers.
The breakthrough emerged with SH3 formed under pressure from H2S, first predicted computationally and subsequently proven experimentally to have Tc = – 70°C at 155 GPa. The structure of SH3 is shown in the figure, where a ReO3 structure is recognized with S in the Re and H in the O sites.
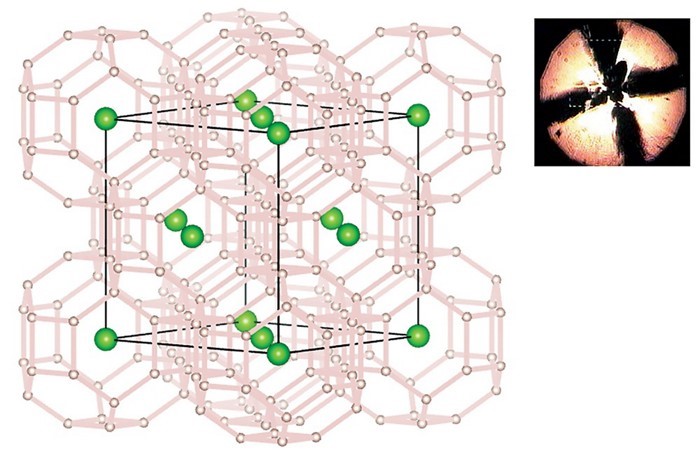
A flurry of activity followed this advance with a periodic table of metal hydride superconductors, which were predicted computationally and verified experimentally. Those with Tc > -70°C include CaH6, YH6, and ThH10 at 100 GPa or higher. Some of these materials have been predicted to be room temperature superconductors, albeit under pressure.
Rare earth hydrogen-rich clathrates are beginning to feature prominently in these investigations, with structures based on covalent hydrogen-hydrogen bonded cages and rare earth atoms occupying the cage centers. An example is the sodalite structure class exemplified by YH10 and LaH10 with H32 cages and predicted Tc = +30°C at 400 GPa and +32-53°C at 250 GPa/+1-13°C at 210 GPa, respectively, figure. LaH10 has been shown experimentally to have Tc in the range of -13 to -23°C.
As things stand today, it appears that simple principles derived from BCS theory, which are guiding massive computational materials searches and reduced to practice experimentally, can show the way to the dream of the room temperature and ambient pressure superconductor.
Geoffrey Ozin
Solar Fuels Group, University of Toronto Ontario, Canada, Email: [email protected], Web sites: www.nanowizard.info, www.solarfuels.utoronto.ca, www.artnanoinnovations.com.
References:
Oganov, Artem R., et al. Structure prediction drives materials discovery, Nature Reviews Materials (2019). DOI: 10.1038/s41578-019-0101-8
Lemonick, Sam. Hunting for the next high-temperature superconductor, ACS Cent. Sci. (2018). DOI:10.1021/acscentsci.8b00817